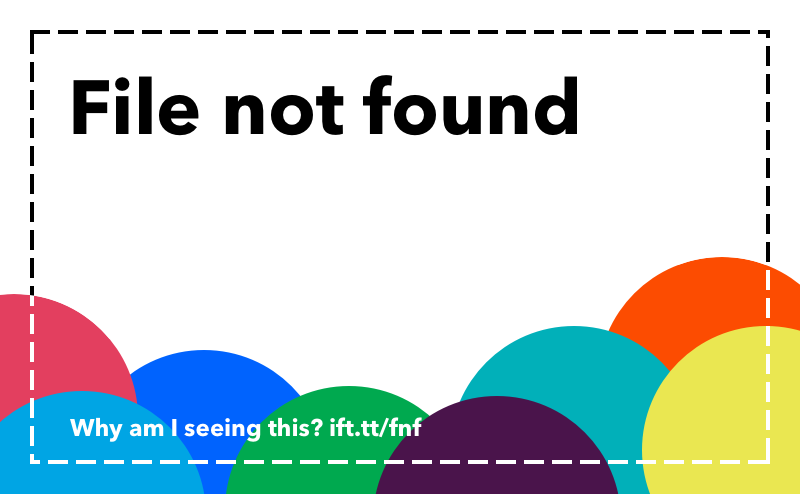
For half a century, climate researchers have considered the possibility of injecting small particles into the stratosphere to counteract some aspects of climate change. The idea is that by reflecting a small fraction of sunlight back to space, these particles could partially offset the energy imbalance caused by accumulating carbon dioxide, thereby reducing warming as well as extreme storms and many other climate risks.
Debates about this idea, a form of solar geoengineering called stratospheric aerosol injection (SAI), commonly focus either on small-scale outdoor research that seeks to understand the physical processes involved or on deployment at a climate-altering scale. The gulf between these is gigantic: an experiment might use mere kilograms of aerosol material whereas deployment that could substantially slow or even reverse warming would involve millions of metric tons per year—a billionfold difference in scale. Appreciably cooling the planet via SAI would also require a purpose-built fleet of high-altitude aircraft, which could take one or two decades to assemble. This long lead time encourages policymakers to ignore the hard decisions about regulating deployment of SAI.
Such complacency is ill-advised. The barrier between research and deployment may be less distinct than is often assumed. Our analysis suggests a country or group of countries could conceivably start a subscale solar geoengineering deployment in as little as five years, one that would produce unmistakable changes in the composition of the stratosphere. A well-managed subscale deployment would benefit research by reducing important uncertainties about SAI, but it could not be justified as research alone—similar research could be carried out with a much smaller amount of aerosol particles. And it would have a non-negligible impact on the climate, providing as much cooling as sulfur pollution from international shipping did before the recent cleanup of shipping fuels. At the same time, the magnitude of the cooling would be small enough that its effects on climate, on a national or regional scale, would be very difficult to detect in the face of normal variability.
While the climate impact of such a subscale deployment would be small (and most likely beneficial), the political impact could be profound. It could trigger a backlash that would upend climate geopolicy and threaten international stability. It could be an on-ramp to large-scale deployment. And it could be exploited by fossil fuel interests seeking to slow the essential task of cutting emissions.
We oppose near-term deployment of solar geoengineering. In accord with the Climate Overshoot Commission, the most senior group of political leaders to examine the topic, we support a moratorium on deployment until the science is internationalized and critically assessed, and until some governance architecture is widely agreed upon. But if we are correct that such subscale deployments are plausible, then policymakers may need to confront solar geoengineering—its promise and disruptive potential, and its profound challenges to global governance—earlier than is now widely assumed.
Obstacles to early deployment
Humans already emit a huge quantity of aerosols into the troposphere (the turbulent lowest layer of the atmosphere) from sources such as shipping and heavy industry, but these aerosols fall to Earth or are removed by rainfall and other processes within about a week. Volcanic eruptions can have a more lasting effect. When eruptions are powerful enough to punch through the troposphere into the stratosphere, the aerosols deposited there can endure for roughly a year. SAI would, like the largest volcanic eruptions, inject aerosols or their precursors into the stratosphere. Given their vastly longer atmospheric endurance, aerosols placed there can have a cooling impact 100 times larger than they would if emitted at the surface.
Getting aerosols to the stratosphere is another matter. Passenger jets routinely reach the lower stratosphere on transpolar flights. But to get efficient global coverage, aerosols are best deployed at low latitudes, where the stratosphere’s natural overturning circulation will carry them poleward and thus distribute them worldwide. The average height of the top of the troposphere is about 17 kilometers in the tropics, and models suggest injection needs to be a few kilometers higher than that to be captured in the upwelling stratospheric circulation. The altitude for efficient deployment is commonly assumed to be at least 20 kilometers, nearly twice the height at which commercial jets or large military aircraft cruise.
Although small spy planes can cruise in this very thin air, they can carry only one to two metric tons of payload. That would be insufficient except for small-scale tests: offsetting a substantial fraction of global warming—say, 1 °C of cooling—would require platforms that could deliver several million metric tons per year of material to the stratosphere. Neither rockets nor balloons are suitable for hauling such a large mass to this high perch. Consequently, full-scale deployment would require a fleet of novel aircraft—a few hundred in order to achieve a 1 °C cooling target. Procuring just the first aircraft in the manner typical of large commercial or military aircraft development programs might take roughly a decade, and manufacturing the required fleet would take several years more.
But starting with full-scale deployment is both imprudent and unlikely. Even if we are turning the global thermostat down, the faster we change the climate, the higher the risk of unforeseen impacts. A country or group of countries that wishes to deploy solar engineering is likely to appreciate the political and technical benefits of a slower start, one with a gradual reversal of warming that facilitates optimization and “learning by doing”, while minimizing the likelihood and impact of unintended consequences.
We envision scenarios where, instead of attempting to inject aerosols in the most efficient way near the equator, a country or group of countries attempt to place a smaller amount of material in the lower stratosphere at higher latitudes. They could do this with existing aircraft, because the top of the troposphere slopes sharply downward as you move away from the equator. At 35° north and south, it is found at roughly 12 kilometers. Adding a 3 kilometer margin, an effective deployment altitude at 35° north and south would be 15 kilometers. This remains too high for airliners but is just below the 15.5 kilometer service ceiling of top-of-the-line business jets made by Gulfstream, Bombardier, and Dassault. The list of countries with territory at or near 35° north or south includes not only rich countries such as the US, Australia, Japan, South Korea, Spain, and China, but also poorer ones such as Morocco, Algeria, Iraq, Iran, Pakistan, India, Chile, and Argentina.
Subscale deployment
How might subscale deployment be accomplished? Most stratospheric scientific studies of aerosol injection assume the operative material is sulfur dioxide (SO2) gas, which is 50% sulfur by mass. Another plausible option is hydrogen sulfide (H2S), which cuts the mass requirement almost in half, though it is more hazardous to ground and flight crews than SO2 and thus might be eliminated from consideration. Carbon disulfide (CS2) gas cuts the mass requirement by 40% and is generally less hazardous than SO2. It is also possible to use elemental sulfur, which is the safest and easiest to handle, but this would require a method of combusting it on board before venting or the use of afterburners. No one has yet done the engineering studies required to determine which of these sulfur compounds would be the best choice.
Using assumptions confirmed with Gulfstream, we estimate that any of its G500/600 aircraft could loft about 10 kilotons of material per year to 15.5 kilometers. If highly mass-efficient CS2 were used, a fleet of no more than 15 aircraft could carry up 100 kilotons of sulfur a year. Aged but operable used G650s cost about $25 million. Adding in the cost of modification, maintenance, spare parts, salaries, fuel, materials, and insurance, we expect the average total cost of a decade-long subscale deployment would be about $500 million a year. Large-scale deployment would cost at least 10 times as much.
How much is 100 kilotons of sulfur per year? It is a mere 0.3% of current global annual emissions of sulfur pollution into the atmosphere. Its contribution to the health impact of particulate air pollution would be substantially less than a tenth of what it would be if the same amount were emitted at the surface. As for its impact on climate, it would be about 1% of the sulfur injected in the stratosphere by the 1992 eruption of Mount Pinatubo in the Philippines. That well-studied event supports the assertion that no high-consequence unknown effects would occur.
At the same time, 100 kilotons of sulfur per year is not insubstantial: it would be more than twice the natural background flux of sulfur from the troposphere into the stratosphere, absent unusual volcanic activity. The cooling effect would be enough to delay global rise in temperature for about a third of a year, an offset that would last as long as the subscale deployment was maintained. And because solar geoengineering is more effective at countering the rise in extreme precipitation than the rise in temperature, the deployment would delay the increasing intensity of tropical cyclones by more than half a year. These benefits are not negligible to those most at risk from climate impacts (though none of these benefits would necessarily be apparent due to the climate system’s natural variability).
We should mention that our 100 kilotons per year scenario is arbitrary. We define a subscale deployment to mean a deployment large enough to substantially increase the amount of aerosol in the stratosphere while being well below the level that is required to delay warming by a decade. With that definition, such a deployment could be several times larger or smaller than our sample scenario.
Of course no amount of solar geoengineering can eliminate the need to reduce the concentration of greenhouse gases in the atmosphere. At best, solar geoengineering is a supplement to emissions cuts. But even the subscale deployment scenario we consider here would be a significant supplement: over a decade, it would have approximately half the cooling effect as eliminating all emissions from the European Union.
The politics of subscale deployment
The subscale deployment we’ve outlined here could serve several plausible scientific and technological goals. It would demonstrate the storage, lofting, and dispersion technologies for larger-scale deployment. If combined with an observational program, it would assess monitoring capabilities as well. It would directly clarify how sulfate is carried around the stratosphere and how sulfate aerosols interact with the ozone layer. After a few years of such a subscale deployment, we would have a far better understanding of the scientific and technological barriers to large-scale deployment.
At the same time, subscale deployment would pose risks for the deployer. It could trigger political instability and invite retribution from other countries and international bodies that would not respond well to entities fiddling with the planet’s thermostat without global coordination and oversight. Opposition might stem from a deep-rooted aversion to environmental modification or from more pragmatic concerns that large-scale deployment would be detrimental to some regions.
Deployers might be motivated by a wide range of considerations. Most obviously, a state or coalition of states might conclude that solar geoengineering could significantly reduce their climate risk, and that such a subscale deployment would strike an effective balance between the goals of pushing the world toward large-scale deployment and minimizing the risk of political backlash.
The deployers could decide that a subscale project might make bigger interventions possible. While scientists may be comfortable drawing inferences about solar geoengineering from tiny experiments and models, politicians and the public may be very cautious about atmospheric interventions that can alter the climate system and affect all the creatures that dwell within it. A subscale deployment that encountered no major surprises could go a long way toward reducing extreme concerns about full-scale deployment.
The deployers could also claim some limited benefit from the subscale deployment itself. While the effects would be too small to be readily evident on the ground, the methods used to attribute extreme weather events to climate change could substantiate claims of small reductions in the severity of such events.
They might also argue that the deployment is simply restoring atmospheric protection that was recently lost. The reduction in sulfur emissions from ships is now saving lives by creating cleaner air, but it is also accelerating warming by thinning the reflective veil that such pollution created. The subscale scenario we sketched out would restore almost half of that sunshade protection, without the countervailing air pollution.
The deployers might also convince themselves that their action was consistent with international law because they could perform deployment entirely within their domestic airspace and because the effects, while global, would not produce “significant transboundary harm,” the relevant threshold under customary international law.
The governance implications of such a subscale deployment would depend on the political circumstances. If it were done by a major power without meaningful attempts at multilateral engagement, one would expect dramatic backlash. On the other hand, were deployment undertaken by a coalition that included highly climate-vulnerable states and that invited other states to join the coalition and develop a shared governance architecture, many states might be publicly critical but privately pleased that geoengineering reduced climate risks.
SAI is sometimes described as an imaginary sociotechnical scenario residing in a distant sci-fi future. But it is technically feasible to start subscale deployments of the kind we describe here in five years. A state or coalition of states that wished to meaningfully test both the science and politics of deployment may consider such subscale or demonstration deployments as climate risks become more salient.
We are not advocating for such action—in fact, we reiterate our support for a moratorium against deployment until the science is critically assessed and some governance architecture is widely agreed upon. Yet a sound understanding of the interlinked technology and politics of SAI is hampered by the perception that it must start with a significant effort that would substantially slow or even reverse warming. The example we’ve outlined here illustrates that the infrastructural barriers to deployment are more easily overcome than is commonly assumed. Policymakers must take this into account—and soon—as they consider how to develop solar geoengineering in the public interest and what guardrails should be put in place.
David W. Keith is a professor of geophysical sciences and founding faculty director of the Climate Systems Engineering initiative at the University of Chicago.
Wake Smith is a lecturer at the Yale School of Environment and a research fellow at the Harvard Kennedy School.
We thank Christian V. Rice of VPE Aerospace for performing the payload calculations herein. Please consult this PDF for more detail on our estimates.
Comments
Post a Comment